See an issue? Let us know!
If you find a mistake or unclarity in this book, please let us know via the lightbulb at the top of the page.
Life#
The origin of life#
One only needs to glance out the window to see the vast variety of life on display. The tree bears no resemblance to the bird, which itself is very different from the mushroom. But our eyes only see a, rather small, fraction of the true diversity. Hidden from view, the soil contains teeming masses of fungi, animals and insects and, throughout it all, between, on and even inside all these organisms are single-celled organisms. The bacteria, archaea and yeasts whose sheer diversity dwarfs all others. Yet this diversity is, to some extent, a matter of perspective. Zoom in far enough and we start to see similarities again. All organisms are constructed of the basic unit of life, the cell. But there is even greater similarity in the processes occurring inside the cell, the biochemistry. Biochemistry is the molecular knowledge of life. All organisms can be simplified as a collection of biochemical processes. While there is a staggering diversity in organisms, at the biochemical level there is a lot more similarity. A lot of pathways, and the molecules involved in them, are the same between single-celled bacteria, plants or large animals. This similarity is a direct consequence of evolution.
This book is about biochemistry. It builds on your previous knowledge of (organic) chemistry and topics like redox reactions and pH. We will delve deeper into those chemical processes that make life unique and allows cells to flourish.
The chemistry of life is not simple. In fact, one of the defining characteristics of life is its chemical complexity. The diversity found in molecules, the multistep pathways that synthesize (or degrade) these molecules. There are circular reaction schemes where at the end of the cycle the original reactant is formed again. Cells are a flurry of activity and organized chaos, none of which would be possible without the ubiquitous biocatalyst, the enzyme.
Another characteristic is the ability to extract, transform and use energy from the environment. Many of the essential biochemical pathways are endothermic and thus cells require a constant influx of energy to keep these pathways running. In plants, this means using the energy provided by sunlight to enable chemical reactions resulting in the formation of small sugars. Other organisms (animals, fungi and many bacteria) oxidize these sugars and other organic compounds to obtain their energy. Bacteria, showing their diversity, have species that rely on the sun (cyanobacteria), the oxidation of organic compounds or which are even able to extract chemical energy from small inorganic molecules (such as hydrogen sulphate or thiosulphate). Another important hallmark of life is its ability to sense and respond to their environment. While some of our senses do not directly rely on biochemistry, many do. It is easy to see that our sense of smell and taste have their basis in chemistry, but perhaps more surprisingly so does sight. We can only tell a photon has reached our eyes when it interacts with the proteins there, for example rhodopsin, and causes an isomerization reaction. Finally, and very importantly, life needs to be able to self-replicate and self-assemble. The basis for this is our genetic material, DNA. DNA needs to be copied, transcribed, translated and proteins self-assemble into the correct shape. But this replication has to not be almost perfect. Small mistakes are necessary for keeping the motor of evolution going, which itself is necessary to adapt to new and changing environments.
So, given all this complexity, how did life start? The current theory is that it started with small inorganic molecules that were around. biochemistry. This theory was proposed in the 1920s by Oparin and Haldane. The theory is that roughly 4-4.5 billion years ago there were shallow seas containing water, carbon dioxide, carbon monoxide, hydrogen, nitrogen. Note that oxygen is not part of this list. Molecular oxygen only appeared after the emergence of photosynthesis. When energy was added to this mixture of chemicals, for example by lighting, spontaneously (small) organic molecules were formed (see Fig. 1). They supplied energy in multiple rounds and found that increasingly more complex molecules were formed. The first round formed simple aldehydes and hydrogen cyanide, which in a subsequent round gave rise to small organic molecules like acetic and formic acid as well are urea. After several rounds, amino acids and molecules like lactic acid had been formed. It was later discovered that in the deep sea there are volcanic plumes containing not only carbon monoxide and carbon dioxide, but also hydrogen sulfate. Nowadays, a lot of scientists think the origin of biochemistry lies at these types of plumes. In the 1950s the first experiments were conducted to test this theory. Miller and Urey mimicked the conditions of the primordial shallow seas in a glass set-up. They then added energy using electric sparks. Afterwards, they tested what molecules were present. After one cycle of sparks, they found small organic molecules such as aldehydes and hydrogen cyanide had formed. After another cycle, small organic acids were formed as well as nitrogen containing compounds like urea. After the third cycle, amino acids were formed. This showed that it was possible to generate these organic molecules from simple molecules and energy. But life needs more than single molecules. The next required step is called condensation, where two molecules form a polymer. This is called condensation because a water molecule is removed. For example, two molecules of glucose make a disaccharide. This process is reversible, upon reaction with water you once again get the two monomers. Condensation as well as its counterpart hydrolyzation is integral to biochemistry and in effect life. Subunits or metabolites condensate into macromolecules like RNA/DNA and proteins. Many of the macromolecules cluster together to form macromolecular assemblies like chromatin or ribosome. The first step, the condensation, is covalent bonds. The second step, the clustering, is non-covalent bonds. We will touch on both of these clustering mechanisms in later chapters.
Fig. 1 A schematic showing a Miller-Urey set up, in a closed system the prebiotic world can be simulated. By introducing energy in the form of an electric spark, it can be tested what types of molecules are created. Retrieved from Wikimedia Commons, licensed under CC-BY-SA.#
Life is not static, but always evolving. We can look at the life that exist now and how they are related to each other. This is often done on the basis of biochemical structures. This information is often given as a phylogenetic tree. There are many ways to construct such a tree, for example through archaeological evidence, through DNA sequence or through the amino acid sequence of proteins. Fig. 2 shows you can see the three major classes of life: bacteria, archaea and eukaryote. This tree was constructed based on sequence of ribosomal RNA. Archaea are a special class of single-cell organisms. They generally are extremophiles living in extreme conditions. They have characteristic changes in molecules in their membranes.
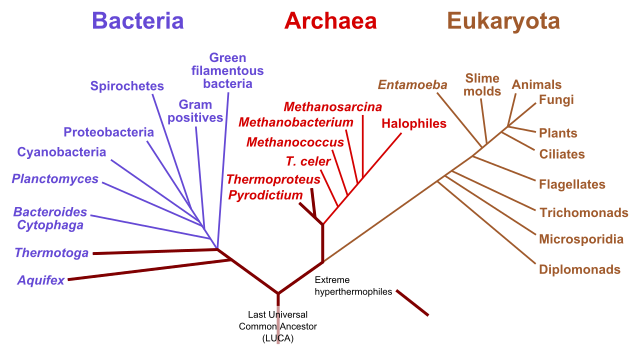
Fig. 2 phylogenic tree showing the bacteria, the archaea and the eukaryota. Adapted from Wikimedia Commons, Public Domain.#
The cell#
The smallest functional unit of life is the cell. At their most basic, cells are a volume (the cytoplasm) enclosed by a barrier (the membrane and, optionally, the cell wall) which contains genetic information, proteins and organic molecules (see Fig. 3). The first recorded observations of the cell can be attributed to two men in the 17th century. The first is the British Robert Hooke, who studied the structure of corks and saw the dead plant cells. The other is the Dutch Antoni van Leeuwenhoek, a cloth merchant from Leiden who build incredibly high-quality microscopes and was able to observe the first live cells. After these discoveries it took another century before cell theory was developed. This was developed by three people: Schleiden, Schwann and Virchow in 1838. The theory states that all organisms are composed of one or more cells and that cells are the basic unit of structure and organization of organisms. Finally, a very important observation was made that cells arise from cells. This established that there was no spontaneous generation, which was still a competing theory at the time.
Fig. 3 Two simplified cells, one animal (left) and one plant (right). This figure shows that at the fundamental level cells have a lot of similarities, but also that there are still significant differences between types of organisms. Retrieved from Wikimedia Commons (left, right), licensed under CC-BY 4.0.#
Cells themselves have a set structure. They contain metabolites: sugars, nucleic acids, amino acids and lipids. These can polymerize to form the next order of structures: fibers (e.g. cellulose or keratin), DNA and proteins. These polymers can be further organized into supramolecular complexes. These are the larger structures in the cell, such as chromatin, the plasma membrane or the cell wall. These larger structures can once again be used to form the large cellular structures called organelles. Examples include the nucleus with the chromosomes, the mitochondria and chloroplasts, as well as others like the Golgi system and peroxisomes. These organelles serve to divide the cell into smaller isolated areas, within which particular conditions can be created.
The chemistry of life#
The complexity of the chemistry of life can be captured by mapping the metabolic processes (see Fig. 4). However, when you study these schematics you can begin to discern patterns. There are long, straight lines where one compound is turned into another, and another and another in a long pathway. Other areas have circles, these are cycles that start and end with the same compound. Many metabolites are involved in these pathways, but some are more important or multipurpose. Acetyl-CoA is one such compound that is a basic building block involved in many pathways. One of these pathways is the citric acid cycle, which we will discuss in detail in a later chapter. Life is not simply a bag of chemical reactions, however. Every point along a pathway is mediated by specialized proteins, the enzymes, and many of these proteins are subject to regulation. How this works will also be discussed in a later chapter.

Fig. 4 A schematic overview of a simplified human metabolism. Each node represents a molecule and each line a conversion. If you study it, you can spot long linear pathways, circular cycles as well as branching points. Retrieved from Wikimedia Commons, licensed under CC-BY 4.0.#
One thing that jumps out when looking at the chemistry of life, is how at first glance it appears to violate the laws of thermodynamics. It is not the first law that is a problem, but the second one. At first, life seems to not obey the law of entropy. For example, plants turn the small molecule CO2 into sugars using energy from light. Entropically this is unfavorable as there is an increase in complexity, this is only possible because of the input of energy from sunlight. The sugars plants produce are used by all other life (including plants themselves). They eat it and through metabolism break down the sugars. This releases energy, part of which is used by the organism (for example to move, grow or propagate) and some is lost as heat. The waste products are again H2O and CO2. The cycle starts with high entropy, low enthalpy molecules (CO2, H2O) and with the input of energy from light high enthalpy and low entropy molecules are produced (sugars). This results in a non-equilibrium steady state, maintained by the constant addition of energy (sunlight) in the biological context we call this steady state: homeostasis. Due to homeostasis, deviations of the steady state are rapidly corrected, a very important concept in biology.
There is another chemical effect that is very important for biochemistry, and which is crucial for its capacity for organization: the hydrophobic effect. If you have a nonpolar solute present in the cell, so present in a watery solution, it will be surrounded by a cage of water molecules. These polar water molecules will surround the nonpolar solute and keep it in solution. When two of these nonpolar solutes come in close proximity, they will coalesce. You can observe this phenomenon when you mix oil and water. A final ingredient in the complexity of cells is how incredibly crowded cells are. There are a lot of structures, macromolecules and metabolites within the cells. This crowding has of course consequences for diffusion. In cells, diffusion is slower compared to regular solutions which allow for the formation of gradient, the importance of which we will discuss in later chapters.
In the coming chapters we will discuss some of the most important classes of biomolecules. Furthermore, we will discuss the pathways and proteins involved in the formation and disassembly of these molecules. We will pay special attention to proteins, their structure, how they work and how they are regulated. Finally, we will discuss how plants are able to capture the energy of sunlight to make sugars, and how we are able to break down these sugars and collect the energy stored within.